Case Studies
UVC Germicidal Disinfection Light Destroys Covid, SARS and other diseases
Covid virus is not unique and is similar to other coronaviruses such as Severe Acute Respiratory Syndrome (SARS) and Middle East respiratory syndrome
(MERS
1. How long does COVID-19 Live on Surfaces? 6 hours – 9 days on surfaces
2. What is the difference between a virus and bacteria?
Bacteria are self-contained, have cell walls, and can survive and replicate on their own.
Viruses are DNA molecules that may be naked or encapsulated and require a host to replicate.
They cannot be treated with antibiotics and require a vaccine
3. What is the biggest issue with this COVID-19 virus? High secondary infection rate, Rapid spread (more rapid than SARS or MERS), Fatality rate (2-
3%)
4. Is ultraviolet light effective against COVID-19?
Ultraviolet light destroys DNA of viruses, bacteria, and molds
The SARS-CoV-2 is a new variant in the beta coronavirus family (Fisher 2020). It transmits by
direct contact or contact with fomites and can be suspended in air as well, as are the related
beta coronaviruses SARS, MERS, and the four known Human coronaviruses – OC43, 229E, NL63, and
HKU1. Most infection transmissions are believed to be by droplet spray from coughing and
sneezing and by direct contact or contact with fomites.
Confirmation That Ultraviolet is Effective
Ultraviolet light can be an effective measure for decontaminating surfaces that may be contaminated by
the SARS-CoV-2 virus by inducing photo dimers in the genomes of microorganisms. Ultraviolet light has
been demonstrated to be capable of destroying viruses, bacteria, and fungi in hundreds of laboratories
studies (Kowalski 2009). Tests on related coronaviruses, including the SARS coronavirus, have
concluded that they are highly susceptible to ultraviolet inactivation.
It is estimated that the SARS-CoV-2 virus can survive on surfaces for up to 9 days.
COVID-19 is highly contagious and so any residual contamination, no matter how small, can pose a threat
to healthcare workers and patients.
Scientific Rationale
Coronaviruses are members of the Corona viridae group and contain a single-stranded, positive-sense
RNA genome surrounded by a corona-like helical envelope (Ryan 1994). Approximately 100 sequences
of the SARS-CoV-2 genome have been published and these suggest there are two types, Type I and
Type II, of which the latter came from the Huanan market in China while the Type I strain came from an
unknown location (Zhang 2020). The genome consists of 29,751 base pairs (NC_045512.2) and the
genome is about 80% homologous with SARS viruses (NCBI 2020, Fisher 2020). Coronaviruses have a
size range of 60-140nm, with a mean size of 0.10 microns (Zhu 2020).
Table 1 summarizes the results of studies that have been performed on Coronaviruses under ultraviolet
light exposure, with the specific species indicated in each case. The D90 value indicates the ultraviolet
dose for 90% inactivation. Although there is a wide range of variation in the D90 values, this is typical of
laboratory studies on ultraviolet susceptibility. The range of D90 values for coronaviruses is 7-2410 J/m2
and the average of all studies is 237 J/m2. However, the study by Walker (2007) is an airborne study and
is an outlier in this set of water-based studies. Also, the studies by Weiss (1986) and Darnell (2004) are
outliers on the low and high ends. Excluding outliers, the mean D90 is 47 J/m2, and this should
adequately represent the ultraviolet susceptibility of the SARS-CoV-2 (COVID-19) virus.
Two recent studies on SARS-CoV-2 have been added to Table 1 (Inagaki 2020, Bianco 2020). The average value of the D90 is 27 J/m2, which suggests the average value for all coronaviruses reported
above (47 J/m2) is conservative. Both studies indicate tailing in the survival curve above about 3-
6 logs of reduction, beyond which the D90 value will not be an accurate predictor (Blatchley 2020).
J/m2 UV k m2/J Base Pairs Kb Source
Coronavirus 6.6 0.35120 30741 Walker 2007a
Berne virus (Coronaviridae) 7.2 0.32100 28480 Weiss 1986
SARS-CoV-2 (Italy-INMI1) 12.3 0.18670 29811 Bianco 2020
Murine Coronavirus (MHV) 15.0 0.15351 31335 Hirano 1978
SARS Coronavirus (Frankfurt 1) 16.4 0.14040 29903 Eickmann 2020
Canine Coronavirus (CCV) 28.5 0.08079 29278 Saknimit 1988b
Murine Coronavirus (MHV) 28.5 0.08079 31335 Saknimit 1988b
SARS Coronavirus (CoV-P9) 40.0 0.05750 29829 Duan 2003c
SARS-CoV-2 (SARS-CoV-2/Hu/DP/Kng/19-027) 41.7 0.05524 29811 Inagaki 2020
Murine Coronavirus (MHV) 103.0 0.02240 31335 Liu 2003
SARS Coronavirus (Hanoi) 133.9 0.01720 29751 Kariwa 2004d
SARS Coronavirus (Urbani) 2410 0.00096 29751 Darnell 2004
Average 237 0.00972
Average excluding outliers 47 0.04943
Average for SARS-CoV-2 27 0.08528
a (Jingwen 2020) b (estimated) c (mean estimate) d (at 3 logs)
Table 1:
REFERENCES
1. Armellino D, Walsh TJ, Petraitis V, Kowalski W. (2019). Assessment of focused multivector ultraviolet
disinfection with shadowless delivery using 5-point multisided sampling of patient care equipment without
manual-chemical disinfection. Am J Infect Control 47,409-414.
2. Armellino D GK, Thomas L, Walsh T, Petraitis V. (2020). Comparative evaluation of operating room terminal
cleaning by two methods: Focused multivector ultraviolet (FMUV) versus manual-chemical disinfection Am J
Infect Contr (Accepted).
3. ASHRAE. (2020). ASHRAE Resources Available to Address COVID-19 Concerns. (American Society of
Heating, Refrigerating and Air-Conditioning Engineers, Atlanta, GA).
4. Bianco A, M Biasin, G Pareschi et al. (2020). UV-C irradiation is highly effective in inactivating and
inhibiting SARS-CoV-2 replication. medRxiv preprint doi:
https://doi.org/10.1101/2020.06.05.20123463 (unreviewed preprint).
5. Blatchley ER, Petri B, Sun W. (2020). SARS-CoV-2 UV Dose-Response Behavior. International Ultraviolet
Association (IUVA) White Paper.
PurpleSun Inc
21-21 41st Ave, Suite 5B, Long Island City, NY 11101
212-500-08595
6. Darnell MER, Subbarao K, Feinstone SM, Taylor DR. (2004). Inactivation of the coronavirus that induces
severe acute respiratory syndrome, SARS-CoV. J Virol Meth 121,85-91.
7. Duan SM, Zhao XS, Wen RF, Huang JJ, Pi GH, Zhang SX, Han J, Bi SL, Ruan L, Dong XP. (2003). Stability
of SARS Coronavirus in Human Specimens and Environment and its Sensitivity to Heating and Environment
and UV Irradiation. Biomed Environ Sci 16,246-255.
8. Eickmann M, Gravemann U, Handke W, Tolksdorf F, Reichenberg S, M€uller TH, Seltsam A. (2020).
Inactivation of three emerging viruses – severe acute respiratory syndrome coronavirus, Crimean–Congo
haemorrhagic fever virus and Nipah virus – in platelet concentrates by ultraviolet C light and in plasma by
methylene blue plus visible light. Vox Sanguinis 115:146-151.
9. Fisher D, Heymann D. (2020). Q&A: The novel coronavirus outbreak causing COVID-19. BMC Med 18,57.
10. Hirano N, Hino S, Fujiwara K. (1978). Physico-chemical properties of mouse hepatitis virus (MHV-2) grown on
DBT cell culture. Microbiol Immunol 22,377-90.
11. Inagaki H, A Saito, H Sugiyama, T Okabayashi, S Fujimoto. (2020). Rapid inactivation of SARSCoV-
2 with Deep-UV LED irradiation. bioRxiv preprint doi:
https://doi.org/10.1101/2020.06.06.138149. (Unreviewed preprint).
12. Jingwen C, Li L, Hao W. (2020). Review of UVC-LED Deep Ultraviolet Killing New NCP Coronavirus Dose.
In Technology Sharing. (Hubei Shenzi Technology Co., Ltd.
13. Kariwa H, Fujii N, Takashima I. (2004). Inactivation of SARS coronavirus by means of povidone-iodine,
physical conditions, and chemical reagents. Jpn J Vet Res 52,105-112.
14. Kowalski W, Bahnfleth W, Raguse M, Moeller R. (2019). The Cluster Model of Ultraviolet Disinfection
Explains Tailing Kinetics. J Appl Microbiol 128,1003-1014.
15. Kowalski WJ. (2009). Ultraviolet Germicidal Irradiation Handbook: UVGI for Air and Surface Disinfection.
(Springer, New York).
16. Liu Y, Cai Y, Zhang X. (2003). Induction of caspase-dependent apoptosis in cultured rat oligodendrocytes by
murine coronavirus is mediated during cell entry and does not require virus replication. J Virol 77,11952-63.
17. NCBI. (2020). Genome Database https://www.ncbi.nlm.nih.gov/. (
18. Petraitis V PR, Schuetz AN, K. Kennedy-Norris K, Powers JH, Dalton SL, Petraityte E, Hussain KA, Kyaw ML,
Walsh TJ. (2014). Eradication of medically important multidrug resistant bacteria and fungi using PurpleSun
Inc. multivector UV technology. In IDWeek. (IDWeek, Philadelphia, PA.
19. Ryan KJ. (1994). Sherris Medical Microbiology. (Appleton & Lange, Norwalk).
20. Saknimit M, Inatsuki I, Sugiyama Y, Yagami K. (1988). Virucidal efficacy of physico-chemical treatments
against coronaviruses and parvoviruses of laboratory animals. Jikken Dobutsu 37,341-345.
21. Walker CM, Ko G. (2007). Effect of ultraviolet germicidal irradiation on viral aerosols. Environ Sci Technol
41,5460-5465.
22. Weiss M, Horzinek MC. (1986). Resistance of Berne virus to physical and chemical treatment. Vet Microbiol
11,41-49.
23. Zhang L, Yang Y-R, Zhang Z, Lin Z. (2020). Genomic variations of COVID-19 suggest multiple outbreak
sources of transmission. medRIX (preprint).
24. Zhu N, Zhang D, Wang W, Li X, Yang B, Song J, Zhao X, Huang B, Shi W, Lu R, and others. (2020). A Novel
Coronavirus from Patients with Pneumonia in China, 2019. N Engl J Med 382,727-73
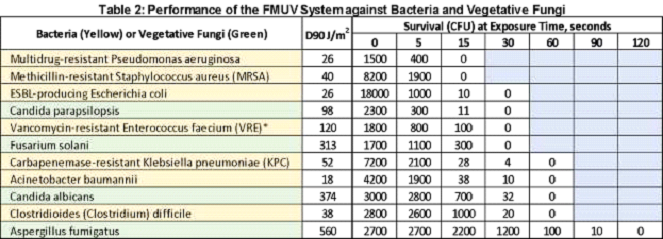
SARS-CoV-2 RNAandSupermarket Surfaces: A Real or Presumed Threat?
1 Department of Biomedical Science and Human Oncology—Hygiene Section, University of Bari Aldo Moro,
Piazza G. Cesare 11, 70124 Bari, Italy; francesco.triggiano@uniba.it (F.T.); francesca.apo@libero.it (F.A.);
giusy.diella@uniba.it (G.D.); marcolopuzzo@gmail.com (M.L.); marilena.dambrosio@uniba.it (M.D.);
fabrizio.fasano1979@libero.it (F.F.); pasquale.stefanizzi@uniba.it (P.S.); osvalda.degiglio@uniba.it (O.D.G.);
mariateresa.montagna@uniba.it (M.T.M.)
2 Department of Prevention, Food Hygiene and Nutrition Service, Local Health Unit BT,
Corso M. R. Imbriani 138, 76125 Trani, Italy; giovanni.sorrenti@aslbat.it (G.T.S.);
pantaleo.magarelli@aslbat.it (P.M.); d.sorrenti2@studenti.uniba.it (D.P.S.);
vincenzo.marcotrigiano@aslbat.it (V.M.)
* Correspondence: giuseppina.caggiano@uniba.it; Tel.: +39-(0)-80-5478-475
Abstract:
Severe Acute Respiratory Syndrome Coronavirus 2 (SARS-CoV-2) emerged in March
2020 in Italy, leading to the pandemic of coronavirus disease 2019 (COVID-19) that continues to
cause high global morbidity and mortality in human populations. Numerous studies have focused
on the spread and persistence of the virus in the hospital setting. New scientific evidence shows
that SARS-CoV-2 is present in different community environments. Although aerosol is one of the
main routes of transmission for SARS-CoV-2, indirect contact through virus-contaminated surfaces
could also play a key role. The survival and persistence of SARS-CoV-2 on surfaces appear to
be influenced by the characteristics of the material, temperature, and humidity. In this study, we
investigated the presence of SARS-CoV-2 RNA on surfaces in 20 supermarkets throughout the Apulia
region during the lockdown period. We collected a total of 300 swab samples from various surfaces
including supermarket scales, trolley handles, refrigerator and freezer handles, and keyboards. In
total, 13 (4.3%) surfaces were positive for SARS-CoV-2 RNA contamination, with shopping trolley
handles being the most frequently contaminated. This study showed that contamination in public
spaces can occur, so we remark the importance to adopt adequate preventive measures, including
environment ventilation, careful surfaces sanitation, hand hygiene, and correct usage of masks, to
reduce the likelihood of virus transmission.
Keywords:
SARS-CoV-2; supermarket; handles; scales; keyboards; surfaces; COVID-19; shopping
trolley handle; refrigeration system handle
1. Introduction
More than a year after the World Health Organization declared the start of the COVID19 pandemic in March 2020, and despite efforts to eliminate the virus, Severe Acute Respiratory Syndrome Coronavirus 2 (SARS-CoV-2) remains a threat to global health. Belonging to Coronaviridae and characterized by positive-sense single-stranded RNA, this virus is responsible for approximately 193,770,895 infections and four million deathsglobally as of 19 July 2021, among which 4,312,673 infections and 127,942 deaths have been in Italy [1]. Although there has never been such a huge amount of information acquired about a disease in such a short time, many aspects of SARS-CoV-2 have yet to be investigated [2,3]. The virus can be transmitted directly from person to person, which is the route most likely to result in infection, but also through other routes, including contaminated surfaces. Nevertheless, the role that surfaces have on the spread of the disease remains debated. Despite common detection of SARS-CoV-2 RNA on surfaces, some studies confirm that the risk of transmission via fomites may be low in clinical settings [4,5].Jones R.M. [6] demonstrated that, when personal protective equipment is not used, droplet and inhalation transmission routes predominate over the contact route, contributing
35%, 57%, and 8.2% of the probability of infection. Some authors report that environmental
contamination by SARS-CoV-2 is high in hospital settings [4,5,7,8] but it is not yet well
known whether asymptomatic individuals can spread the infection in the same way as patients with coronavirus disease 2019 (COVID-19). Surface contamination by asymptomatic
individuals has been demonstrated [9], but data in non-healthcare settings are still minimal.
Harvey et al. [10] estimated risk of infection from touching a contaminated surface to be
low (less than 5 in 10,000). However, it is suspected that indirect contact through viruscontaminated surfaces may also have a role in transmission [11,12]. Besides, environmental
surveillance is an important tool for monitoring infectious disease prevalence, especially
diseases with several asymptomatic subjects, so targeted sampling of high-touch surfaces
can help with other pandemic surveillance strategies in identifying the passage of infected
subjects.
In Italy, SARS-CoV-2 RNA has been detected in molecular investigations of surfaces
in tourist and recreational facilities, such as bathroom door handles, refrigerator handles,
handrails, and bar counters [13]. Although molecular investigation can detect the viral
genome, viability of the virus has not been demonstrated using these methods.
The survival and persistence of SARS-CoV-2 on surfaces appear to be conditioned by
characteristics of the material as well as temperature and humidity. A recent study [14]
showed that UK SARS-CoV-2 strain, England 02/2020 remains viable for longer periods on hydrophobic surfaces (up to seven days) as compared with hydrophilic surfaces
(three days).
The aim of the study was to investigate the presence of SARS-CoV-2 RNA on surfaces in supermarkets to inform which surfaces are most contaminated and require more
attention during disinfection procedures.
2. Materials and Methods
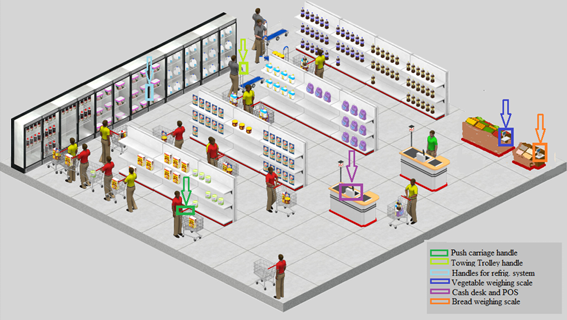
Figure 1. Example diagram of the sampling points. POS, point of sale.
2.2. Environmental Sampling Surface sampling was carried out using sterile swabs inserted into plastic tubes (Easy Surface Checking Swab Neutralizing Rinse Solution, NRS; Liofilchem Srl, Roseto degli Abruzzi, TE, Italy) containing 10 mL of transport medium. Sampling was carried out on flat surfaces by sliding and rotating a moistened swab over a standard-sized sampling area (10 × 10 cm); on non-flat surfaces, any available area (e.g., handles) was sampled. Sampling was carried during time slots that were representative of high levels of customer utilization (between 9 a.m. and 12 a.m.). Samples were transported to the laboratory in a special isothermal refrigerator at a controlled temperature (4 °C) and immediately processed. 2.3. Molecular Analysis To evaluate the method of extraction, amplification/detection, and efficiency recovery, preliminary in vitro studies were conducted on different types of surfaces such as wood, steel, and glass using a strain of Feline Coronavirus (FCoV) type II 25/92, isolated from a cat with infectious peritonitis. The swabs were vortexed for 20 s and transferred to a new 15-mL tube under sterile conditions. We conducted molecular investigation for the presence of SARS-CoV-2 RNA using real-time reverse transcription-polymerase chain reaction (RT-PCR), as documented in the literature and our previous study [13,15]. Nucleic acid was extracted with 5 mL of NRS medium using the NucliSENS miniMAG semi-automatic extraction system with magnetic silica, according to the manufacturer’s instructions (bioMerieux, Marcy-l’Etoile, Lyon, France). To monitor the quality of the extraction procedure, we used a negative control (i.e., a swab with sterile neutralizer) and positive control (RNA extraction of our process control virus, or FCoV). RNA was resuspended in 100 µL of elution buffer and the extracts were stored at −20 °C. For amplification of the ORF-1ab gene (nsp14), a mixture of 25 µL was prepared containing 5 µL of RNA for each sample; 12.5 µL of 2X Reaction Buffer supplied with AgPathID™ One-Step RT-PCR Reagents (Applied Biosystems, Thermo Fisher Scientific, MA, USA); 1 µL of 25X RT-PCR enzyme mix; 1 µL of forward primer (12.5 µM); 1 µL of reverse primer (22.5 µM); 1 mL of probe (6.25 µM); 1.83 µL of nuclease-free water (not DEPC-treated); and 1.67 µL Real-Time PCR Detection Enhancer (Applied Biosystems). The primer and probe sequences used were as follows: CoV-2-F/ACA TGG CTT TGA GTT GAC ATC T; CoV-2-R/AGC AGT GGA AAA GCAT GTG G; CoV-2-P/FAM-CAT AGA CAA CAG GTG CGC TC-MGBEQ [15]. Real-time RT-PCR was conducted in duplicate using the CFX96 Touch Deep Well Real-Time PCR System (Applied Biosystems). Thermal cycling conditions were 50 ◦C for 30 min (reverse transcription phase); 95 ◦C for 10 min (inactivation of the RT phase); 95 ◦C for 15 s; and 60 ◦C for 45 s (45 amplification cycles). For RT-PCR, we used the nuclease-free water supplied by the kit as a negative control and an aliquot of the previously extracted FCoV as a positive control. Cycle threshold (Ct) cutoffs for RT-PCR were used as indicators of the SARS-CoV-2 RNA copy number in the samples, with lower Ct cutoffs corresponding to higher viral copy numbers. A Ct value < 40 was interpreted as positive for SARS-CoV-2 RNA.2.4. Statistical Analyses
We carried out statistical analyses using Fisher’s exact test to compare the different percentages of positivity among swabs with respect to the type of surface analyzed, the category of surface analyzed, and the COVID-19 risk level where each supermarket was located (color coded) to detect any statistically significant differences. Multivariate analysis using the Poisson Regression Model was done to compare results of number of PCR cycles about SARS-CoV-2 surface contamination with respect to the type of surface analyzed, the COVID-19 risk level where each supermarket was located (color coded), and overall data on COVID-19 cases found 14 days before and 14 days after the sampling date in the province of analysis. The choice of the 14 days before and after was identified in such a way as to consider all the possible cases of infection present in the examination area and in the sampling period on the basis of the maximum incubation period of the infection. The data of COVID-19 cases were collected, as well as time of day that the sample was collected [16]. Descriptive parameters were transformed into numbers (ordinal encoding technique), assigning consecutive numbers to each parameter in alphabetical order (e.g., Parameter A = 1, Parameter B = 2, and so on) [17]. To standardize the different units of measurement for the six independent parameters, the data were normalized using the following formula [18]: x normalized = (x − xminimal)/(xmaximum − xminimal); a Poisson regression model was used. All the above parameters were considered in the preliminary model. The possible final model included only those parameters with a p-value < 0.05. To quantify the possible effects of the above parameters on the PCR trend, we calculated the effect (eβ − 1), which corresponds to the relative risk. In both types of analysis, a statistically significant result was considered with p-values < 0.05. We used R version 3.6.3 in the statistical analysis (The R Project for Statistical Computing, Vienna, Austria).3. Results
A total of 300 surface swabs were tested; of these, 13 (4.3%) swabs were positive for SARS-CoV-2 RNA. The shopping trolley handle and scales were the most frequently contaminated among all surface categories (5.0%, respectively), followed by refrigeration system handles (3.8%), and keyboards (3.8%). Among sampling points, ice cream freezer handles and mixed-product freezer handles were the most frequently positive for SARSCoV-2 RNA (14.3%, respectively), followed by shopping trolley handles (10%) and selfservice produce scales (5.9%) (Table 1).Table 1. Analyzed samples, distributed according to category and surface type. In bold we indicate the total positive samples for category.
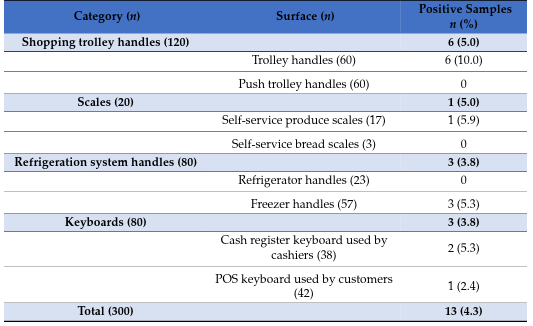
Table 1. Analyzed samples, distributed according to category and surface type. In bold we indicate
the total positive samples for category.
Table 2. Summary of statistically significant results of Fisher’s exact test.

Lorem ipsum dolor sit amet, consectetur adipiscing elit. Ut elit tellus, luctus nec ullamcorper mattis, pulvinar dapibus leo.
4. Discussion
It is scientifically proven that surfaces contaminated with viruses and bacteria can act as a medium for the transfer of microorganisms to the hands, and subsequently, to the gastrointestinal or respiratory tract [19]. With respect to SARS-CoV-2, it has been widely demonstrated that infection with this virus mainly occurs via the respiratory tract [2,3]. However, contact of mucous membraneswithacontaminatedsurface and/orwithdroplets can also lead to infection with SARS-CoV-2 [12]. There are many opportunities for envi ronmental contamination (air and surfaces) with SARS-CoV-2 in hospital settings where COVID-19 patients may be present [4,7,8]. On the contrary, evidence regarding environ mental spread of SARS-CoV-2 in the community is lacking. Pitol et al. [20], evaluated that he risks of community transmission of SARS-CoV-2 through surfaces are low and that the effectiveness of surface disinfection is highly dependent on the infection prevalence and the frequency of contacts. Although it is estimated that the transmission of SARS-CoV-2 via fomites is low, it is possible and can favor the onset of new cases during outbreaks. In our study, we examined different surfaces in supermarkets during periods of limited mobility owing to COVID-19 restrictions. We found the presence of SARS-CoV-2 RNAcontamination in 4.3% of the swab samples analyzed. These results are consistent with other papers in which less than 10% of the swabs were contaminated by SARS-CoV-2 [10]. No significant difference between supermarkets in areas at the two highest (red and orange) risk levels were found. A potential hypothesis to explain this result can be due to the lack of period between the contamination of SARS CoV-2 on surfaces and the real onset of infection or disease. It is important to emphasize that entering supermarkets at these risk levels involved the obligatory use of masks and disinfection/sanitization practices. However, we found that freezer handles and trolley handles were frequently positive in testing for SARS-CoV-2 RNAcontamination. Thehighestcontaminationofthesesurfaces is probably related to the occurrence of use. In fact, they were frequently touched by customers and were all non-porous surfaces with the same ability to retain contamination of SARS-CoV-2. Regarding the shopping trolleys, weobserved a significant contamination of the trolley handles. This result can be correlated with the availability of dispensers for hand sanitation. In our study, in all supermarkets, the hand hygiene dispenser was positioned near the push trolley deposit at the entrance to the supermarket, and the other shopping trolleys were in areas diametrically opposite to the disinfectant dispenser. We can assume that although it was strongly recommended to sanitize hands, not all customers disinfected their hands at the entrance, but rather they immediately went to take the shopping trolleys. Therefore, customers using the push shopping trolleys were more likely to disinfect their hands before using the shopping trolleys because the dispenser was near. These data lead us to underline the importance of more careful sanitization on these surfaces during the cleaning and disinfection procedures. This is essential hand hygiene, so it is necessary to increase the number of sanitation systems in community environments, in particular in proximity to surfaces that are the most frequently touched. Scientific evidence has shown that the presence of SARS-CoV-2 RNA on a surface does not mean that the surface itself is infectious. We found Ct values between 36.88 and 38.63 in our positive samples, i.e., tending toward high values; however, some studies have shown that high values are indicative of partial RNA degradation or low viral load [21,22]. Consequently, we can hypothesize that, despite compliance with precautionary measures, SARS-CoV-2 RNA can circulate in enclosed environments frequented by healthy, perhaps asymptomatic, individuals. These observations do not mean that the surfaces contaminated bySARS-CoV-2RNArepresentahighdangeroftransmission of the virus, in fact the results of our study cannot irrefutably support these theories but can only show the presence of SARS-CoV-2 genetic material, not how much this can be correlated to the danger of infection. The virus can survive on surfaces made of various materials and its degree of infectiv ity can vary from hours to days, depending on the environmental conditions; environments with high temperatures and high humidity can inactivate the virus more quickly [23,24]. SARS-CoV-2 has been shown to survive for up to 4 h on copper surfaces, for up to 24 h on cardboard, and for up to 2–3 days on plastic and stainless steel [11,25]. In China, COVID-19 clusters have been repeatedly linked to imported frozen raw foods. SARS-CoV-2 RNA has been detected on food packaging materials (frozen shrimp packaging), food storage environments (shipping containers), and even some foods (frozen chicken wings) [3]. In this study, we did not analyze the type of material at each sampling point. We were more interested in verifying whether this virus could be found in environments that are frequented by the public during periods of restricted mobility in the COVID-19 pandemic. Int. J. Environ. Res. Public Health 2021, 18, 9404 7 of 9 In terms of both significant and non-significant data, our findings suggest interesting con clusions. We further confirmed that surfaces of public environments can be contaminated by SARS-CoV-2 RNAandwehypothesize that they could be a potential virus transmission vehicle. It is notable that more frequently positive surfaces were those hypothesized to be most often touched by hands. Thus, we highlight the need to pay particular attention to hand and environmental hygiene. Detection of SARS-CoV-2 on surfaces believed to be touched frequently and by many people in a community indicates that increased sanitization and hand hygiene in public spaces, such as grocery stores, may be useful in decreasing SARS-CoV-2 transmission via fomites. During periods with high circulation of SARS-CoV-2 RNA, it is necessary to intensify cleaning management programs, such as by installing disinfectant solution dispensers near high-contact surfaces used by the public [26]. Furthermore, to continue drawing attention to the need for adopting adequate preventive procedures, it is necessary to increase the number of graphic signs in public places, as well as repeated broadcast messages [27]. With a view to the protection and safety of employees, adoption of a specific protocol to avoid the spread of SARS-CoV-2 RNAisneededinstoresfrequented by large numbers of people each day [26]. This is in addition to recent recommendations of the Italian Institute of Health [28]. This study has some limitations. First, we did not isolate the virus from samples to assess viability; we also did not collect air samples or test supermarket customers. Furthermore, more in-depth multivariate analysis of factors with non-significant results is warranted, as well as including other parameters (e.g., size of POS devices, frequency of POSuse, cases occurring specifically in the municipalities where sampled supermarkets are located). In light of the emergence of new viral variants, this is our future aim, using more standardized environmental detection techniques. Moreover, the introduction of quantitative methodologies to search for SARS-CoV-2 on swab samples is another possible development of our research. This methodology would allow us to apply a QMRAmodel[10] to estimate infection risk of the disease on every surface.5. Conclusions
Our study shows that surfaces in non-hospital environments can be contaminated by SARS-CoV-2 RNA. Contamination in public spaces can occur and warrants further investigation to evaluate viability and risk, including collection of behavioral data to understand what surfaces are touched the most often, how often people hand sanitize, and how often they touch their face. Considering that surfaces such as trolleys with handles have been found to be more often contaminated, perhaps it would be appropriate to pay more attention to these surfaces during the sanitization procedures. Certainly, it remains essential to underline the importance of prevention measures such as ventilation of environments, careful hand sanitation, and correct usage of masks in public locations in the community, such as supermarkets, and commensurate with the number of visitors at such locations.
Conflicts of Interest: The authors declare no conflict of interest.
References
1. World Health Organization. Novel Coronavirus (2019-nCoV): Situation Report. Available online: https://www.salute.gov.it/
portale/nuovocoronavirus/dettaglioContenutiNuovoCoronavirus (accessed on 20 July 2021).
2. Han, Q.; Lin, Q.; Ni, Z.; You, L. Uncertainties about the transmission routes of 2019 novel coronavirus. Influenza Other Respir.
Viruses 2020, 14, 470–471. [CrossRef] [PubMed]
3. Lange, C.; Wolf, J.; Auw-Haedrich, C.; Schlecht, A.; Boneva, S.; Lapp, T.; Agostini, H.; Martin, G.; Reinhard, T.; Schlunck, G.
What is the significance of the conjunctiva as a potential transmission route for SARS-CoV-2 infections? Ophthalmologe 2021, 118
(Suppl. 1), 85–88. [CrossRef] [PubMed]
4. Chia, P.Y.; Coleman, K.K.; Tan, Y.K.; Ong, S.W.X.; Gum, M.; Lau, S.K.; Lim, X.F.; Lim, A.S.; Sutjipto, S.; Lee, P.H.; et al. Singapore
2019 Novel Coronavirus Outbreak Research Team. Detection of air and surface contamination by SARS-CoV-2 in hospital rooms
of infected patients. Nat. Commun. 2020, 11, 2800. [CrossRef] [PubMed]
5. Razzini, K.; Castrica, M.; Menchetti, L.; Maggi, L.; Negroni, L.; Orfeo, N.V.; Pizzoccheri, A.; Stocco, M.; Muttini, S.; Balzaretti, C.M.
SARS-CoV-2 RNA detection in the air and on surfaces in the COVID-19 ward of a hospital in Milan, Italy. Sci. Total Environ. 2020,
742, 140540. [CrossRef] [PubMed]
6. Jones, R.M. Relative contributions of transmission routes for COVID-19 among healthcare personnel providing patient care. J.
Occup. Environ. Hyg. 2020, 17, 408–415. [CrossRef] [PubMed]
7. Guo, H.; Li, W.; Huang, Y.; Li, X.; Li, Z.; Zhou, H.; Sun, E.; Li, L.; Li, J. Increased microbial loading in aerosols produced by
non-contact air-puff tonometer and relative suggestions for the prevention of coronavirus disease 2019 (COVID-19). PLoS ONE
2020, 15, e0240421. [CrossRef] [PubMed]
8. Ong, S.W.X.; Tan, Y.K.; Chia, P.Y.; Lee, T.H.; Ng, O.T.; Wong, M.S.Y.; Marimuthu, K. Air, Surface Environmental, and Personal
Protective Equipment Contamination by Severe Acute Respiratory Syndrome Coronavirus 2 (SARS-CoV-2) From a Symptomatic
Patient. JAMA 2020, 323, 1610–1612. [CrossRef]
9. Huang, Y.; Ding, Z.; Chen, Q.; Wu, L.; Guo, L.; Zhao, C.; Sha, L.; Sun, H. Environmental virus detection associated with
asymptomatic SARS-CoV-2-infected individuals with positive anal swabs. Sci. Total Environ. 2021, 753, 142289. [CrossRef]
[PubMed]
10. Harvey, P.A.; Fuhrmeister, E.R.; Cantrell, M.E.; Pitol, A.K.; Swarthout, J.M.; Powers, J.E.; Nadimpalli, M.L.; Julian, T.R.; Pickering,
A.J. Longitudinal Monitoring of SARS-CoV-2 RNA on High-Touch Surfaces in a Community Setting. Environ. Sci. Technol. Lett.
2021, 8, 168–175. [CrossRef]
11. Van Doremalen, N.; Bushmaker, T.; Morris, D.H.; Holbrook, M.G.; Gamble, A.; Williamson, B.N.; Tamin, A.; Harcourt, J.L.;
Thornburg, N.J.; Gerber, S.I.; et al. Aerosol and Surface Stability of SARS-CoV-2 as Compared with SARS-CoV-1. N. Engl. J. Med.
2020, 382, 1564–1567. [CrossRef]
12. Liu, Y.; Li, T.; Deng, Y.; Liu, S.; Zhang, D.; Li, H.; Wang, X.; Jia, L.; Han, J.; Bei, Z. Stability of SARS-CoV-2 on environmental
surfaces and in human excreta. J. Hosp. Infect. 2021, 107, 105–107. [CrossRef]
13. Montagna, M.T.; De Giglio, O.; Calia, C.; Pousis, C.; Apollonio, F.; Campanale, C.; Diella, G.; Lopuzzo, M.; Marzella, A.; Triggiano,
F.; et al. First Detection of Severe Acute Respiratory Syndrome Coronavirus 2 on the Surfaces of Tourist-Recreational Facilities in
Italy. Int. J. Environ. Res. Public Health 2021, 18, 3252. [CrossRef]
14. Paton, S.; Spencer, A.; Garratt, I.; Thompson, K.A.; Dinesh, I.; Aranega-Bou, P.; Stevenson, D.; Clark, S.; Dunning, J.; Bennett, A.;
et al. Persistence of SARS-CoV-2 virus and viral RNA in relation to surface type and contamination concentration. Appl. Environ.
Microbiol. 2021, 87, e0052621. [CrossRef] [PubMed]
15. La Rosa, G.; Mancini, P.; Bonanno Ferraro, G.; Veneri, C.; Iaconelli, M.; Bonadonna, L.; Lucentini, L.; Suffredini, E. SARS-CoV-2has
been circulating in northern Italy since December 2019: Evidence from environmental monitoring. Sci. Total Environ. 2021, 750,
141711. [CrossRef] [PubMed]
16. Statistiche Coronavirus. Available online: https://statistichecoronavirus.it/coronavirus-italia/coronavirus-puglia/ (accessed on
19 August 2021).
17. Potdar, K.; Pardawala, T.S.; Pai, C.D. A Comparative Study of Categorical Variable Encoding Techniques for Neural Network
Classifiers. Int. J. Comput. Appl. 2017, 175, 7–9. [CrossRef]
18. Patro, S.; Sahu, K.K. Normalization: A Preprocessing Stage. IARJSET 2015. [CrossRef]
19. Otter, J.A.; Donskey, C.; Yezli, S.; Douthwaite, S.; Goldenberg, S.D.; Weber, D.J. Transmission of SARS and MERS coronaviruses
and influenza virus in healthcare settings: The possible role of dry surface contamination. J. Hosp. Infect. 2016, 92, 235–250.
[CrossRef]
20. Pitol, A.K.; Julian, T.R. Community Transmission of SARS-CoV-2 by Surfaces: Risks and Risk Reduction Strategies. Environ. Sci.
Technol. Lett. 2021, 8, 263–269. [CrossRef]
21. Matson, M.J.; Yinda, C.K.; Seifert, S.N.; Bushmaker, T.; Fischer, R.J.; van Doremalen, N.; Munster, V.J. Effect of Environmental
Conditions on SARS-CoV-2 Stability in Human Nasal Mucus and Sputum. Emerg. Infect. Dis. 2020, 26, 2276–2278. [CrossRef]
22. Petrillo, S.; Carrà, G.; Bottino, P.; Zanotto, E.; De Santis, M.C.; Margaria, J.P.; Giorgio, A.; Mandili, G. A Novel Multiplex qRT-PCR
Assay to Detect SARS-CoV-2 Infection: High Sensitivity and Increased Testing Capacity. Microorganisms 2020, 8, 1064. [CrossRef]
Int. J. Environ. Res. Public Health 2021, 18, 9404 9 of 9
23. Chin, A.W.H.; Chu, J.T.S.; Perer, M.R.A.; Hui, K.P.Y.; Yen, H.L.; Chan, M.C.W. Stability of SARS-CoV-2 in different environmental
conditions. Lancet Microbe 2020, 1, e10. [CrossRef]
24. Biryukov, J.; Boydston, J.A.; Dunning, R.A.; Yeager, J.J.; Wood, S.; Reese, A.L.; Ferris, A.; Miller, D.; Weaver, W.; Zeitouni, N.E.;
et al. Increasing Temperature and Relative Humidity Accelerates Inactivation of SARS-CoV-2 on Surfaces. mSphere 2020, 5,
e00441-20.
25. Gholipour, S.; Nikaeen, M.; Mohammadi Manesh, R.; Aboutalebian, S.; Shamsizadeh, Z.; Nasri, E.; Mirhendi, H. Severe Acute
Respiratory Syndrome Coronavirus 2 (SARS-CoV-2) Contamination of High-touch Surfaces in Field Settings. Biomed. Environ. Sci.
2020, 33, 925–929. [PubMed]
26. Ministero Della Salute Shared Protocol for Updating Measures for Contrast and Containment of the Spread of the SARS-CoV2/COVID-19 Virus in the Workplace. Available online: https://www.salute.gov.it/imgs/C_17_pagineAree_5383_0_file.PDF
(accessed on 10 June 2021).
27. Zandonadi, R.P.; Botelho, R.B.A.; Maynard, D.D.C.; Akutsu, R.D.C.C.D.A. Self-Service Restaurants in SARS-CoV-2 Pandemic.
Encyclopedia 2021, 1, 401–408. [CrossRef]
28. Draisci, R.; Attias, L.; Baldassarri, L.; Catone, T.; Cresti, R.; Fidente, R.M.; Marcello, I.; Buonanno, G.; Bertinato, L. Raccomandazioni
ad Interim Sulla Sanificazione di Strutture Non Sanitarie Nell’attuale Emergenza COVID-19: Ambienti/Superfici. Aggiornamento del
Rapporto ISS COVID-19 n. 25/2020. Versione del 20 Maggio 2021. (Rapporto ISS COVID-19, n. 12/2021); Istituto Superiore di Sanità:
Rome, Italy, 2021